The Importance of Dog Bones and Doughnuts: Revisiting the Freeluna Space Program
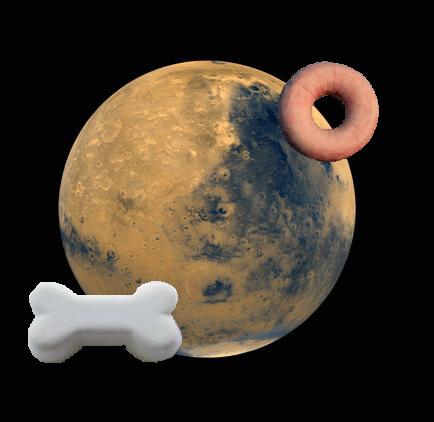
Given the success of SpaceX and Elon Musk's and Mars One's intent to colonize Mars, I think it's high time to revisit the notion of a rotating space station in low earth orbit, and eventually using said station (or something like it) to transport people to Mars. Before we go colonizing other planets, we really need to find out if humans can stay healthy in a partial gravity (less than 1 g but more than 0 g) environment, such as what humans will encounter on Mars (0.377 g) or the Moon (0.165 g). To the best of my knowledge, there has been absolutely no research done in these two gravity regimes. Our knowledge of zero gravity (microgravity) is extensive, and we have known for decades that exposure to it causes substantial physiological decay. What we need to find out is how badly or if Mars colonists will suffer decay within the gravity field of Mars.
To this end, let me introduce you to the concepts used to design a rotating space station created for the purpose of testing partial gravity fields on the human body. In the old 50's sci-fi movies, we saw plenty of examples of spinning wheels in space that represented space stations. In the movie 2001: a Space Odyssey, Dr. Heywood R. Floyd first finds himself heading for a double wheeled space station spinning in Low Earth Orbit, before he heads to the moon. The principle of artificial gravity used in these films is just an application of conservation of angular momentum, commonly called centrifugal force. Spinning a person around in circles can cause problems such as dizziness for some people, but almost all people can tolerate a spin rate of 2 rpm and some people can tolerate a spin rate as fast as 7 rpm. Depending on the RPM and the gravitational pull one is trying to induce, the radius of the spinning space station varies. The formula for calculating the radius for earth-like gravity is as follows:

Where 9.8 is acceleration of gravity at Earth's surface in meters/second^2 (use 3.7 for Mars and 1.6 for the moon), 'pi' is 3.14159.., and so on. With this calculation in hand, you can derive various habitat radii for different RPM.

If one so desired, a apparatus could be set-up on earth to simulate these spin rates and radii to test for human spin tolerance, such as with a vehicle connected to a central pivot via appropriate length cables and forced to drive around in circles to simulate station spin. The induced gravitational pull would be somewhat over 1 g, but should allow observation of inner ear phenomena, nonetheless. A graph below shows the various speeds of such a system...

Building a complete space station ring could get quite expensive, but building just a pole with small habitats on either end of it would be far less of a challenge. For a Mars equivalent gravity in a station spinning at 2 RPM, the length of the pole would need to be about twice the radius shown in the first table, or about 169 meters long. This could be reduced by offsetting the center of mass of the station towards one end of the pole. By placing most of the mass of the station at one end of the pole, the pole length to the Mars habitat would still need to be 84.4 meters from the center of mass of the station, but the pole length extending the opposite direction could be much shorter. The extra mass could consist of the station's batteries, rocket engines, fuel, water, solar, panels, radiators, etc. It might also contain a lunar habitat, using the shorter radius of 36.5 meters, thus allowing testing of both Martian and Lunar gravity effects in one station. A crude layout of such a station, which I am calling "the Dog Bone", is shown below:
The martian habitat would be on the left end of the station's central boom, and the lunar habitat would be on the right end of that boom. The extra pod to the right of the lunar habitat represents the extra weight of various station components which offsets the station's Center of Mass or Center of Gravity (I'm using the terms interchangeably here. Sorry, sue me if you must.). If it can be shown that subjects can tolerate a higher RPM, then the central boom of the station shrinks significantly.
I don't think such a station need be incredibly expensive to build. The central boom is the most awkward piece of the station, and I believe it could be assembled in orbit. The boom is, after all, not much more than a big aluminum pipe. Assuming 3 boom sections could be put in orbit per flight, with each boom being approximately 13 meters long, it would take about 3 flights just for the boom sections, potentially two more flights for habitat sections, and two more flights for the "counterweight" and docking interface, so somewhere around $480 million for launch costs for the station. Assuming my numbers are off by a factor of three, this is still a very inexpensive space project, and the payoff for having such a station is huge.